SEAVOLUTION - MEGA FLOATS FOR A SUSTAINABLE FUTURE
S.H. Lim1 and C.M. Wang2
1Managing Director, EMAS Consultants LLP, Singapore
2Director, Engineering Science Programme, National University of Singapore, Singapore
Earth is a planet of incredible beauty. Even with the Hubble Space Telescope, scientists cannot find another planet that comes close to Earth’s richness in diversity. While the other planets in our solar system look barren and even threatening, ours when seen from outer space is covered by a soft hue of blue. It is just right for the evolution of life that has taken the single-celled organism to modern man.
Equipped with a disproportionately big brain, man has become very successful at adapting his environment for his security, food and his well-being. He has no predator and has so far overcome all manners of diseases that threaten his specie. He is however, a threat to all other forms of life around him.
His superior intelligence, paradoxically, has degraded the planet, threatening his food supply, poisoning the water he drinks and the air he breathes. His ability to live longer than all but a few mammals has increased his demand for food and water. Yet scientists are attempting to prolong his life vastly longer. In the US alone, US$2.4 billion is spent annually by the government on research to stop ageing. The pressure on Earth’s resources is expected to mount.
The natural world has deteriorated. The technological advances man has made since he discovered fossil fuel is largely the cause. This degradation is the largely the result of the atmosphere having risen in temperature, possibly 2 degree Centigrade limit set a few years ago, thanks to the relentless pursuit for economic growth. The temperature rise destabilises the global climate, causing the polar caps and glaciers to melt, snow caps on mountains to retreat, and weather patterns to change.
A POPULATION CRISIS
The current world population growth is about 1.2% per annum. This growth rate is untenable for long. Even if it were to slow it down to 0.5%, it would grow exponentially at 147 times in a 1000 years (1.0051000=146.57) taking the population to more than a thousand million people. Can this planet sustain a thousand million people? Look what we have done to it with just 7 billion people.
Population distribution weighs heavily against Asia. With about a third of the world’s land mass; it has to support 60% humankind. Its population also grew at the fastest rate, by about four times in the last century. Singapore is a tiny country, which has the dubious distinction of being the most densely populated country in the world, with a population that has grown three times in the last 50 years.
Man is harming his environment, “contributing to a loss of biodiversity, deforestation and loss of topsoil, and shortages of water” (IAP); in short, putting his kind in peril. If we pollute the Earth further, beyond a tipping point, the point of irreversibility, we risk our own extinction. The most intelligent being on the planet, ironically will be displaced by the insect, a life form that has survived his abuse.
Rich nations, in particular the US are spending substantial resources to explore other planets in our solar system (as well as extrasolar planets so called exoplanets) as an alternative habitat to Earth.
Assuming another asteroid does not hit this planet, as one did 65 million years ago, in 500 years, at a reduced growth rate, say 0.5% per annum, there will be 85 billion people to feed, and to share the world’s water resources. 500 years is not an eternity. A similar time span would take one back to the time when the first Europeans came to Asia. The world population then was about 500 million.
To put things in perspective, the Earth is estimated to be about 4.5 billion years old. Dinosaurs dominate the planet for 135 million years before the fallout from an asteroid exterminated them. Homo sapiens on the other hand have been around not more than 200,000 years. Yet he is already heading to his extinction. It is highly unlikely that modern man, will survive longer than one percent of the time he has been around, if world population continues to grow at the present rate. Because by then, planet Earth may not be able to produce all the energy, food and clean water that he needs.
Wackernagel (1994) calculated the ecological footprint (i.e. the area of the planet’s surface required to support the population’s lifestyle with water, energy, food and resources and waste absorption) and showed that the hectares of land per capita are shrinking as shown in Fig. 1.
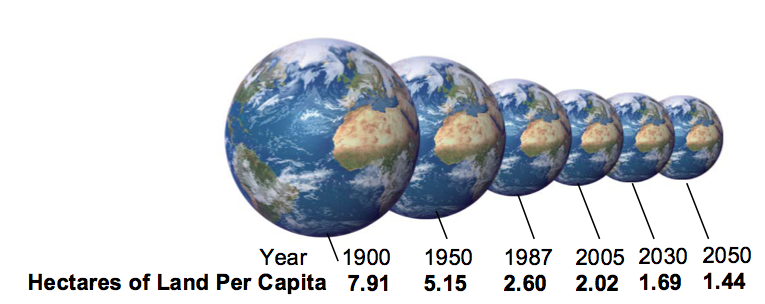
SEAVOLUTION
The sea is man’s last frontier. It is borderless and should remain that way for so long as man walks on this planet.
Seavolution is about living sustainably perhaps for the next half-millennium at least. It is a blueprint for a fresh start: of living with Nature and not against Nature, allowing the land and the skies to recover from the wanton abuse of man.
Man’s love affair with fossil fuel must be curtailed. Seavolution provides the best means available to reduce that fatal bond. Seavolution is about supplementing the food and energy resources of land with bounties from the sea. It is about the sea as a source of energy, food and clean water.
The sea, about 2.3 times as big in area as land and which spreads out in three dimensions rather than two, is humanity’s reserve tank. Much of the planet’s land mass is barren. The farmable land is less than 9% of total land mass. The sea is a resource we need to put to use. The land that has served us tirelessly needs a break.
BOUNTIES OF THE SEA
The resources that man needs to keep him alive are clean water, clean air, clean energy and uncontaminated food. These can no longer be taken for granted. In pursuing economic growth, man has depleted these resources to a dangerous level. The good news is that the sea has all of these. Wisely used, these resources may yet sustain us for several centuries; but, only if we live harmoniously with it. That is to say, if we renounce our wanton disregard for nature and if we respect it as the people of the jungle respect every tree and creature in it.
Supplier of Oxygen
More oxygen comes from the sea that from all the vegetation on the land. In fact the ocean accounts for 50 to 85 percent of the oxygen in Earth’s atmosphere largely through phytoplanktons, the microorganisms that whales feed on. Like plants on land they convert carbon dioxide to oxygen using the sun’s energy. As currents circulate sea water globally, so does these life-giving planktons (the Greek word for drifters).
Remover of Carbon Dioxide
The ocean has played a major role in absorbing the increased carbon dioxide due to human activities such as burning fossil fuels for energy and production of cement for construction. Figure 2 shows the effects of more carbon dioxide on our planet Earth.
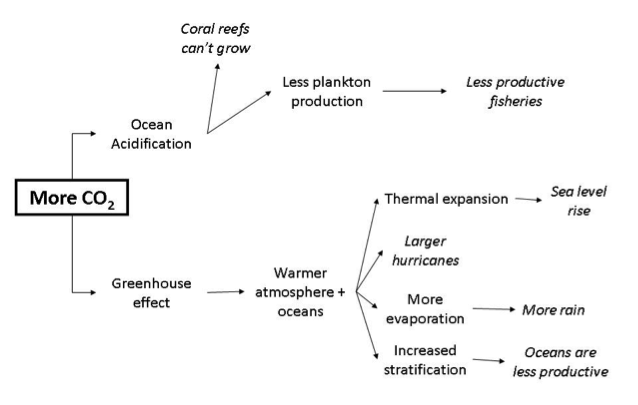
Source of Water
All the water we drink originates from the sea. From the sea, we get water vapours, clouds, rain and snow. Water keeps the entire planet alive and returns to the sea (hopefully detoxified) to start the next cycle.
While desalination of seawater is already a commercial process, that process is largely driven by fossil energy. Pure solar desalination, which depends on the sun’s energy and nothing else, is still a long way from mass production. Not being commercially viable is one reason. The other being where water is most needed there is not sufficient land space and a decent water source to produce clean water. If there was, it is mostly put to use for the production of salt rather than water, because salt is in demand and is able to fetch a better price than water.
Very large floating platforms may be a game changer, as we shall see later in this paper.
Source of Food
The sea has supplied man with food since ancient times. The sea makes no demand on man in return for the food it provides. Fish does not compete with man for water and grain as do livestock on land. It is estimated that to produce one kilogram of beef, 15.4 cubic metres of water is required, and for one kilogram of pork, 6 cubic metres of water is needed.
Food from the sea includes edible weeds, which grow rapidly and require neither fertiliser nor pesticide. The sea does not require ploughing.
The extraction of food from the sea, be it fish or weed, leaves little carbon or water footprint. Any means to improve the production, and increase the output of seafood to supplement land-cultivated food is a step towards making the world a greener place.
An average of “2,000 hectares of irrigated land in arid and semi-arid areas across 75 countries have been degraded by salt” every day for the past 20 years, according to a study by UN University’s Canadian-based Institute for Water, Environment and Health. If plants can be genetically modified to process saline water, we would solve the world’s food shortage. And there is. “Scientists have found a way of genetically modifying cereal crops to grow in salty water” according to a report in the Telegraph.
Source of Energy
While the oceans have abundant deposits of oil and gas (many yet to be discovered), it is also our best hope for a source of renewable energy. It is estimated that the solar energy that impacts the Earth, in the form electromagnetic waves is 10,000 times what is needed by humanity. About 43.5% of the global solar irradiance is absorbed by land and oceans of our planet. As the oceans of our planet constitute about 71% of the earth’s surface area, a larger portion of the absorbed solar energy goes into the oceans. At present, the most abundantly used energy sources are fossil fuels but the world energy consumption is only 0.005% of the global solar irradiance (see Fig. 3). The continuous burning of fossil fuels for energy could result in catastrophic global warming effects. It is therefore imperative that ways of developing unlimited sources of new energy that do not result in greenhouse gases and global warming be found and implemented. One such approach is to mine the abundant resource of solar energy that remains untapped in the oceans via Ocean Thermal Energy Conversion (Wang et al., 2001). Because the ocean is so huge, extraction of that energy will have only a minute effect on the environment.
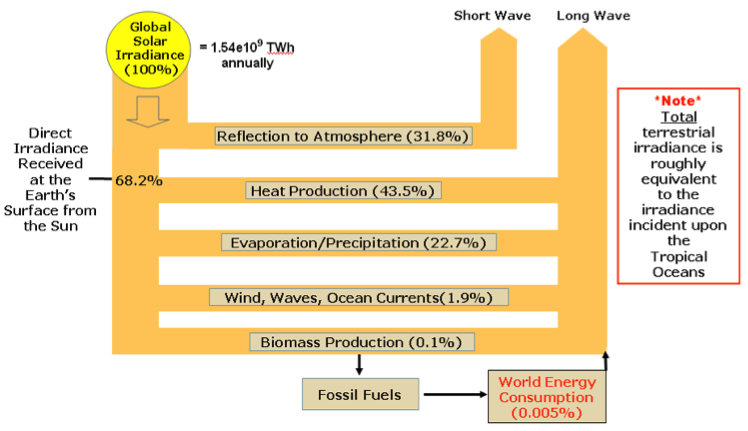
Hitherto, man has stuck to the easy way of using the energy of the sun that has been stored under underground and seabed in the form of coal, oil and gas. He is only beginning to learn to tap the wind, sun’s ray, ocean currents and ocean thermal gradients. The oceans are also awash with marine vegetation that may be converted to biofuel.
MEGA FLOATS FOR A SUSTAINABLE FUTURE
Although the world comprises 148,940,000 square kilometres of land, most of it is uninhabitable deserts, mountains or snow-covered tundra. The 45 million square kilometres that is cultivated or used as farms for livestock, provide sustenance for 7 billion people as well as their habitat. This works out to 156 people per square kilometre or about half the size of a football field for each inhabitant. This share would reduce to a quarter of the size of a foot field when the population doubles in about 55 years (at the present 1.2% growth rate per annum) and rising sea levels, due to global warming, inundate more low lying coastal and riverine land particularly in Asia and flat islands in the oceans.
The Israelis have succeeded in turning deserts into fertile land for cultivation of food. Mountains have been terraced into rice fields. However there are limits to what is possible. We cannot farm the snowfields of Antarctica or the tundras of Canada. While billions of dollars have been invested to search for life in outer space, the potential that the ocean offers remains largely neglected.
The ocean offers opportunities that few have yet realised. A simple solution to many of the world’s problems is to create very large floating structures (VLFS) or floating islands, to farm the bounties and diversities of the sea, to harvest the rain that falls on it, and tap the enormous amount of clean energy it receives from the sun. VLFS technological development has reached a stage where such notions have moved from the stuff of fantasy to imminent reality (see Wang et al. 2008 and Wang and Wang 2014). Although an unfortunate start (unfortunate, because in the ultimate analysis, fossil fuel is bad for all life forms), we are already extracting oil and gas from deposits beneath the seabed. The technology that has enabled rigs to stay afloat and keep their position under high winds and waves suggests a solution to harvest the sea for clean energy, water and food sustainably without the collateral damage.
United Nations Registry of VLFS in international waters
The sea is not marked by rivers and mountain ranges, which on land mark national boundaries. The 12 nautical mile limit agreed by the UN Convention on the Law of the Sea is necessary for jurisdictional and defence reasons. Beyond that limit, there is no valid physical or historical reason that any nation can bring to bear to support a claim of ownership for any area of the sea. It is hoped that world leaders will respect the doctrine of freedom of the sea.
All nations including land locked ones should have access to the sea and to use the sea for peaceful and ecologically acceptable purpose. These are high ideals but one a rational world community needs to uphold. A possible scenario would be as follows. The ownership of a floating structures moored in international waters must traceable to a legal person who is a national of a member country of the United Nations.
The UN shall maintain a registry of such assets and administer them under a new agency similar to the IMO (referred hereafter for convenience as IMO2). The legal regime applicable on the float shall be that of the member country under which it is registered (flagged). IMO2 is tasked with licensing floating structures in international waters. To apply or renew their license, owners need to subject their asset, periodically to the scrutiny of international inspectors appointed by IMO who shall certify the non-military nature of the asset. They shall also certify that the floats are seaworthy and comply with rules to protect human lives at sea and all forms of marine life in their vicinity. (Note that the IMO already has a set of comprehensive rules such as SOLAS and MARPOL applicable to ships).
Underwriters would require the owners of these assets to validate their license as a condition for validation of insurance policies just as they require seaworthy certificates for ships.
Engineering solutions
The first semi-submersible came into being in in 1962 when it commenced drilling an oil well 297 feet beneath the waters of the Gulf of Mexico. In a little over 50 years, engineers have delivered an array of floating offshore rigs operating in waters, some more than 2500 metres deep and working in extreme weather conditions such as in the North Seas where waves can be higher than 20 metres and wind can gust at more than 50 knots. Most floaters are of the semi-submersible design comprising ballast tanks submerged below the sea surface thereby reducing the action of large wave and wind and improving stability.
What spurred the rapid advances in the design and construction of oil rigs was the escalating oil prices, which went from below US$20 in 1998 to US$140 in 2008. Funds for new research both in the commercial sector and in the universities were seldom in short supply. The findings of these research and analysis quickly find their way to support each new generation of offshore rigs. These findings are well documented in engineering journals around the world as well as in design rules to be found in the books of the classification societies such as the American Bureau of Shipping, Lloyds Register of Shipping and Det Norske Veritas. Unfortunately, although there is a wealth of knowledge, mainly in the academia, for the design of VLFS for non-oil and gas applications, so far no attempt has been made to compile them into a rulebook like the Euro Code for practitioners.
However even without a specially tailored code of practice such as the Euro Code, it is possible to draw on both the rules of the classification societies and those of Euro Code (or other national codes) to produce a coherent and seaworthy structural design.
The mechanical and electrical system would require to lean more heavily for marine practice. Unless nearshore, a floating complex in general needs to have an on-board power generation plant which needs to be rated for operation in a marine environment. This would mean having a substantial reserve of fuel oil for between refills. Assuming a specific fuel consumption of 0.25kg/kWhr, a 1 Megawat plant running 24 hours consumes 6 tons of fuel. If the refill frequency is 30 days, a tank capacity of 180 tons is needed. Noise and gas emissions need to comply with marine standards.
Firefighting and ventilation requirements need to satisfy IMO standards. All outdoor electrical systems need to be of marine grade to endure the humid saline atmosphere. The mechanical and electrical systems on a large cruise ship should provide many helpful ideas and solutions. These are covered by the rules of the classification societies for passenger ships.
Platform construction
A major consideration in constructing a mega platform is the launching of the structure. If the platform is too large to be launched as a single unit, it will need to be fabricated in several blocks or modules. Each module is then launched separately, floated, manoeuvred, aligned and connected to the rest of the modules to form the final whole. Each module should be as large as possible to reduce the number of joints. The joining may be done at the place of fabrication or at the destination.
A shipyard with a dry dock (either graving or a floating dock) or a launch way would be recommended for the construction and launching of blocks with a length longer than 100 meters, particularly if it was made of concrete. Shipyards have tugboats and experienced tradesmen to rig, tow and connect the modules. There are many shipyards in China, Korea, Japan, Philippines, Vietnam and Singapore with the necessary facilities and capability to undertake such work. Already a consortium of Japanese shipyards has built a 1000-metre long floating trial runway in Tokyo bay (see Fig. 4).
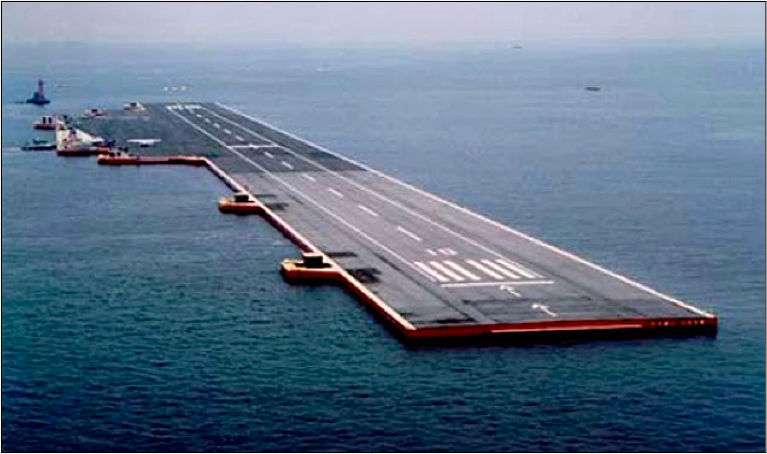
Smaller floats, of steel construction and hence lighter, may be built on an open beachfront and launched using air bags, or in a temporary dock with earth bund cofferdams, which are removed to flood the dock when the floats are ready for towing to site.
Superstructure erection
Terrestrial buildings are built from ground upwards, beginning with the columns then the beams and followed by the joist. As the building rises, these elements have to be lifted piece by piece usually by just one or two tower cranes. Following a few floors behind are the mechanical and electrical fixtures, they too have to lift by crane. Workers have to commute vertically from ground to whatever floor they happen to be working. When the elevator breaks down, construction work stalls. Inclement weather hampers progress.
With a floating building, shipyard production methodology may be employed. A 20-storey building would be divided into four 5-storey blocks, each with its own work area, served by its own crane. The construction of all four block is carried out in parallel, under roof. Columns, beams, joists, ventilation ducts, power and communication cables, pipework for wastewater, firefighting and drinking water commence at each of the four blocks in parallel, at low heights. No worker needs to climb higher than 20 metres on any day. Likewise, no material needs to move more than a few floors.
If each floor requires a 10-day cycle to complete, the building would require 200 days to erect floor-by-floor from ground to the top not counting days lost due to bad weather. A building under roof divided into four blocks can be fabricated in 50 days with no days lost due to bad weather. Supervision would be easier because of the low level at which the work takes place and hence higher quality may be expected.
Each of the four blocks would be rolled out of the workshop, lifted by a floating crane. There are several floating cranes in Asia with capacities ranging from 1000 to 5000 tons. A lifting and joining operation, common in large shipyards, would not take more than a day for each block. Lifting points are carefully assessed and approved before skilled riggers set about their work. Steel buildings lend themselves better to such erection methods than concrete ones. In any case, steel is the preferred material for the superstructure as they are lighter per unit area of floor space, which translate into smaller buoyancy requirements.
It is worth noting that cruise ships, which are as luxuriously outfitted as five-star hotels are likewise constructed in blocks, and outfitted before the blocks are joined. This is very unlike the procedure by which the famous Titanic was built. The Titanic was built in a fashion similar to the way buildings on land are built: piece by piece, plate by plate from the keel upwards.
Towing
Towing if over short distances is easily accomplished with tugboats. However, floats may be towed anywhere in the world from China to Singapore or the Middle East. Figure 5 shows the fully assembled Concrete Island Drilling System (CIDS) being towed by two 22,000 horsepower tugs from Japan to the Beaufort Sea, North of Alaska. For long distance towing, “dry tow”, are generally necessary. In a dry tow the payload transported on the deck of a special submersible vessel. The riggings are approved and certified seaworthy by competent bodies before the voyage begins.
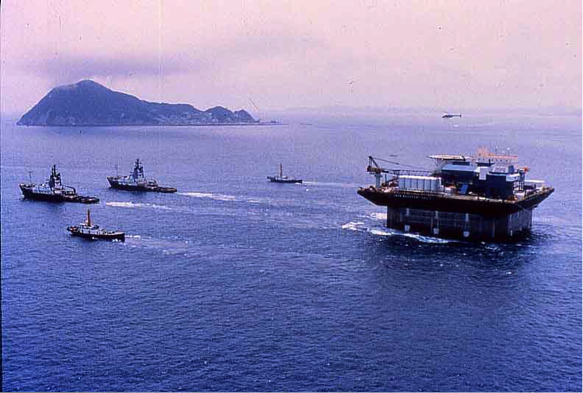
Underwriting
Large floating complexes require considerable financing. Bankers are part of the investment equation. To be bankable, all phases of the project from design to construction, delivery, towing and securing the float at site have to be based on sound engineering principles. While floating assets such as described are a new field of endeavour, the application of proven practices in the marine, offshore oil and gas are valid. Together with the stamp of approval of a renowned classification society, underwriters should have the necessary level of comfort to endorse such project. The risks associated with floating buildings, seaports, airports, incinerators, golf courses etc. are not any greater than those to which offshore oil rigs are exposed and in fact considerably less. Furthermore, observations have indicated that the chances of a terrestrial building perishing in an earthquake or a tsunami or simply the failure of its foundation are higher than one erected on a floating platform.
The fact that a floating asset is a movable asset lessens the risk of an investment. If a business fails in one economy, the same business may be re-sited in another where the business environment may be more favourable. For example a floating shipyard facing problems with high labour cost in Singapore have the option to operate in Indonesia simply by towing it across the border, or sell the entire asset to someone in the Philippines. There are no such options to exit the business for a shipyard built on land. The major assets of a land shipyard are immovable civil engineering structures. When the shipyard has to close, the landowner (as is the practice in Singapore) requires reinstatement of the property to its original condition. This means all civil engineering structure below and above ground have to be demolished.
URBANISATION OF THE SEA
The sea provides the urban town planner a space of unrestricted possibilities: clean slates to plan a metropolis, where he can let his imagination take hold. There are no difficult terrains he has to avoid, no geological challenges that prevent tall buildings to be erected, nor nature reserves around which highways have to circumvent.
Although the sea is more forgiving than land (it allows structures to be nudged, or moved after they have been built and moored), it is nevertheless necessary to zone the sea into plots each with its own specific building requirements. This is so that over the years as the town or city develops, it is orderly and efficient and the seascape is pleasing to the eye, and not one chaotic floating jungle.
The sea offers some significant advantages. For one, no highways need to connect floating towns. A town can be constructed in any part of the sea without the developer having to worry that the landowner may run out of funds to build roads leading to his town and throwing asunder his cash flow projections. Likewise, the urban planner need not concern himself with highways and roads, or even with water and power.
The capacity of a floating platform to support a load and its inherent stability against toppling over can be calculated precisely based on the principles of physics. This is not necessarily so with terrestrial buildings especially if they require deep foundations. There are far too many unknowns and unknowables in the geology of any site. Many buildings have collapsed because of poor interpretation of soil data obtained from the field or the imprecise science of soil mechanics.
Rejuvenating waterfront land
Many cities are characterised by their waterfront skyline. New York, Sydney, San Francisco, Hong Kong and Singapore are good examples. Waterfront assets command a premium. They are showcases of a nation because of their high visibility. Sadly, not all cities realise this. Many waterfronts are marred by depressing warehouses, ports, shipyards, power stations, tank farms, petrochemical plants. Others, like in Jakarta are sinking because of both soft ground and rising sea level.
Mega floats provides an unique opportunity for coastal cities to (a) increase their stock of waterfront space and (b) to relocate facilities such as incinerators, power plants, ports, naval bases, wholesale fish markets far out at sea away from the residential and urban environment without denying the them the waterfront that they need.
To create waterfront space, clusters of floating islands of concrete or steel may be moored some distance, 500 to 1000 metres away from existing shorelines. On these may be built parks, apartments, offices, cruise and convention centres similar to the manmade Marina Bay in Singapore. Marina Bay is built on a piece of land reclaimed about 30 years ago. Today it would be possible for such assets to be built on a cluster of floating platforms.
Mega platforms would make large space possible for exclusive abodes for resort living such as Sentosa Cove in Singapore (see Fig. 6). It took many years for the reclaimed land in Sentosa Cove to consolidate before construction could begin. With floats, the time needed would be reduced significantly.
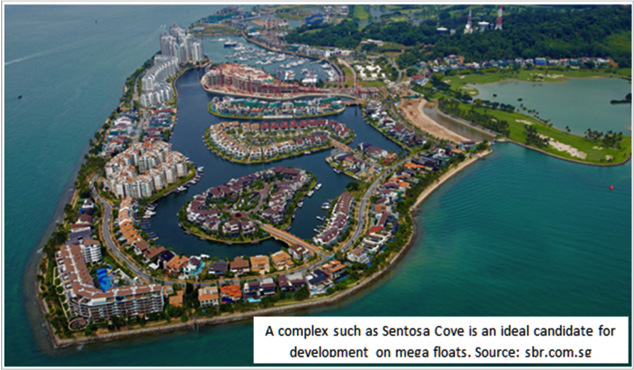
A recent advertisement offers a 4-bedroom apartment at the Cove for SGD 13 million. The 99-year leasehold land alone was valued at “S$1,612.90 per square foot (land)” about USD 13,800 per square metre. A float with a designed life of 100 years could be built in a low cost environment, like Indonesia for example, and delivered to Singapore for a tenth of that price.
Certain facilities, of which shipyards, naval bases and seaports are examples, have to be beside the sea. In the past, it was taken for granted that the only location for them was either on river banks or coastal land or land reclaimed from the sea. Today with VLFS, there is the additional option of locating such facilities on floating islands some distance away from the shoreline. Each case merits special analysis. The urban planner and the user should address these questions: Can the land that is currently occupied by a shipyard, naval base or a port be put to a better use as the city expands to cope with a growing population? Can these facilities benefit from being on a floating island? Would their operational effectiveness be diminished if they were not onshore?
Figure 7 shows how several floats may be assembled to form a larger floating platform for a shipyard for the repair and maintenance of VLCC and offshore rigs.
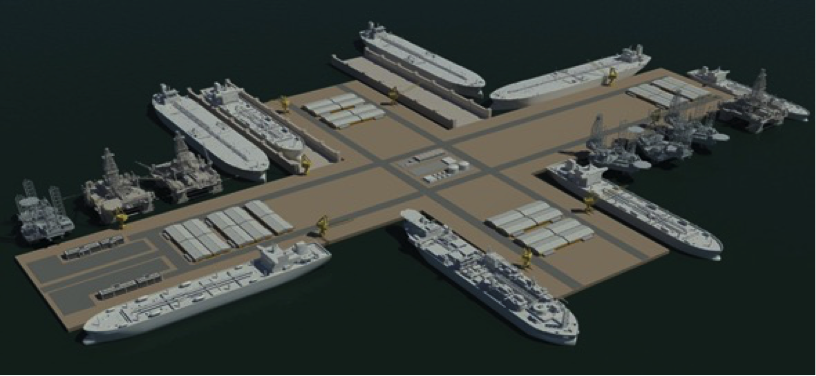
To increase waterfront needed for ship repairing, the configuration may be in the shape of the letters H, E, or L or t as shown in Fig. 7. For shipbuilding where more land relative to the berths is needed, a rectangle may be a more efficient layout. In this example floating docks provide the dry docking capability. This solution may solve the land scarcity problem for shipyards wishing to expand their operation and at the same time wishing to be ready to relocate to lower cost centres in the region when things get tough.
An article published in Today Newspaper on 14 January 2015, entitled “No more land in Singapore? Build floating real estate” is appended at the end of this paper. Singapore is island of about 720 sq. km. If the population growth rate continues, it won’t be long before the overcrowding becomes unbearable. The city-state has no hinterland and any space needed for a growing population several decades from now, will have to be created by VLFS. Reclamation of land is neither an economical nor an environmentally sensitive solution. Land prices in Singapore ranges from USD 14 to 15,000 per square metre; thereby making the construction cost of a VLFS looks very attractive in comparison.
Floating skyscrapers
How high can building be when built on a floating platform? It can be as high as buildings on land, provided that the water depth is sufficiently deep. Shimizu, a leading Japanese contractor has announced their concept of a 1-km high floating city; 72 meters higher than Burj Khalifa (see Figure 8). See this link for details: http://www.shimz.co.jp/english/theme/dream/greenfloat.html
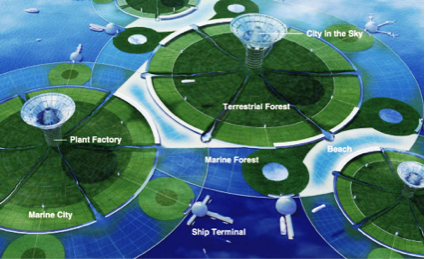
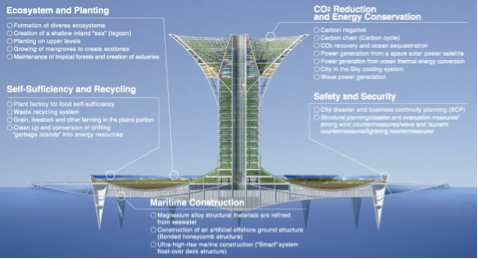
Figure 8: Shimizu’s floating city in the sky
While the Burj Khalifa needs a foundation of 8000 square metres with 192 piles each 50 metres long topped with 3.7 metres concrete cap to carry its 500,000 ton mass, the offshore processing plant, Prelude FLNG is capable of carrying a higher load (600,000 tons) just floating on water and in far worse conditions of wind and waves. The Prelude is 488 metres long and 74 metres wide (see Fig. 9). It requires a single mooring point to keep its position above the seabed, which lies 240 metres below it.
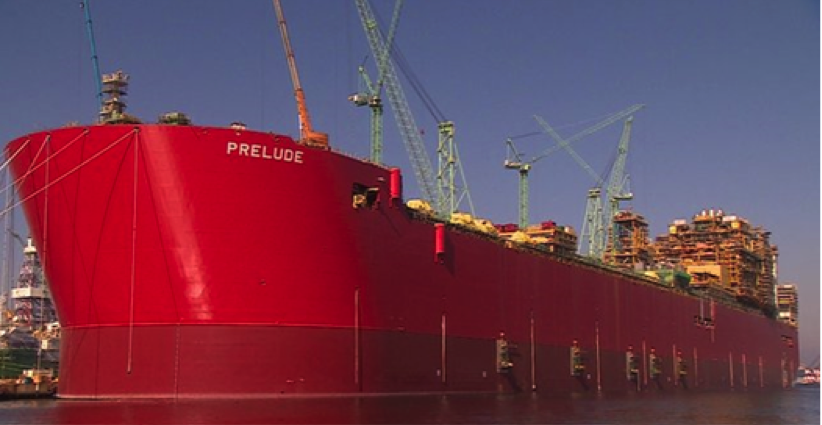
How safe is a floating structure in a typhoon prone area? Large ships (including cruise ships) in harbour and at sea have encountered typhoons but none has been known to succumb in recent years. A search on the internet confirms this. Figure 10 shows a typical situation of an offshore floating accommodation unit keeping its position in a raging storm. This one uses a 12-point anchoring system. A floating structure on a large platform has better stability than a ship.
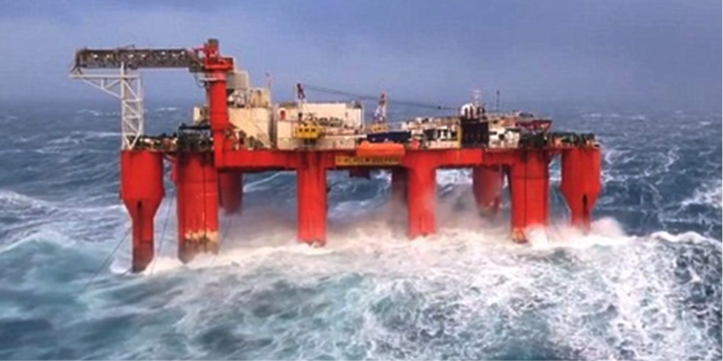
Recycling VLFS
Over time, a facility becomes obsolete, because its original function has been overtaken by changes in the market place (shipyards in Japan face this problem), or rapid technological advances requires major redesign of the structure. Whatever the reason, facilities often become dated before the designed life of the structure in which they are housed.
What does one do with a structurally sound platform after its original function ceases to be economically relevant? It is not difficult to envisage other possible uses for the platform or even the building on top of it. It could serve a useful purpose in a less advanced economy. An old floating office tower may be turned into a floating school in Papua New Guinea. An old floating logistic warehouse in Japan may find a new lease of life as a garment factory in Philippines. There will be no lack of takers for used floating structures in a world with such disparity in economies.
At some stage, a structure may be too old and unsound even for a third world country. How does one dispose of a floating asset? The answer is a lot easily than a terrestrial asset. No demolition is necessary. After removing all potentially harmful materials, these structures may be sunk in the ocean. The BBC files this story about the relationship between marine life and shipwrecks. It shows clearly the benign nature of shipwrecks and, by extension VLFS, abandoned at the bottom of the sea.
Of course, if it makes economic sense, VLFS can be towed to a shipyard and be broken down so that the building material (steel, aluminium, copper, glass) may be recycled. This would be more efficient than demolishing a terrestrial building in a city and clogging up city streets with trucks carting debris away. Shipbreaking provides employment for thousands in countries such as India, Pakistan and Bangladesh.
The floating shopping mall
Shopping malls are ubiquitous features of modern city living. Every new city boasts at least one. What about floating cities? Would it be possible to have a shopping mall with hundreds of retail outlets, restaurants, cinemas, theatres, bars, nightclubs, hotels, swimming pools, and playgrounds constructed on a VLFS? Yes, of course. What more, it moves. It comes to your neighbourhood instead of you having to go to it.
A 400 metre long, 100 meter wide floating mall with 12 decks is a feasible proposition. It would have a gross floor area of 480,000 square metres with easily 300,000 square metres of revenue generating space. It may be fitted with azipods to enable it to manoeuvre safely around floating islands and to remain dynamically “fixed” anywhere in the sea without casting anchors in areas where anchors may cause damage to mooring devices of the floating islands. It would have landing jetties for small boats and ferries. Hovercrafts or amfibuses shuttle between the islands and the floating mall transporting visitors. These watercrafts can be driven on board and visitors embark and disembark as easily as stepping on and off a transit system. RO-ro seatrucks deliver vans and 20-foot containers with goods to replenish the stores. The vans roll on and off the floating mall, while containers are lifted by ship cranes.
It would provide a new life style. More importantly, it would take thousands of vehicle-trips off terrestrial roads, reduce road maintenance and pollution and eliminate the need to provide car parking spaces in towns.
Highways without concrete and steel
Waterborne vehicles require no highways, viaducts or tunnels. The enormous national savings in not having to build and maintain them should be factored into the urban planner’s strategy.
Yes, they still burn fuel, but solar powered electric engines have more chance of being a mass transport reality on water than on roads. However, watercrafts are more eco-friendly. The fuel they consumed per passenger-mile is significantly less than that for cars, buses and trucks. With more space on board watercrafts, much more efficient exhaust gas scrubbers than those fitted in road vehicles may be installed. Gas emission at sea is dispersed more rapidly than on roads. Outdoor air pollution kills more than 3.5 million people across the world every year, with transport accounting for more than half, according to an OECD report. Less air and noise pollution translate to savings in the national healthcare budget, which again has to be taken into account in evaluating the financial viability of floating cities.
In low-density traffic situations, which will prevail for several years from the commencement of urbanising the sea, no more need be done other than mark shipping lanes with buoys. In future, the authors foresee all water borne crafts will be beaming signals every second of their location to a control centre for watercrafts, just like aircrafts sending signals to air control towers. A computer will then take over all the piloting of the craft for the entire journey, so efficiently that even grandma can go meeting her friends alone with her boat. She would not be a target of a road rage as well as it would not be possible for someone to jump off his boat and get physical.
For longer distances, floating airports can be built. They are no more sophisticated than the docks in a marina. No money is needed to build or maintain the runways. Seaplanes take off and land on a very short stretch of water. What is needed is a floating jetty to park the seaplanes and for passengers to board and disembark. In Canada and the US, it is a common way of getting around for coastal cities. A remotely operated seaplane is not inconceivable given the fact that in the worst scenario, it is possible to land safely in an area the size of a football field in the sea.
Google, Amazon and DHL currently develop drones. Safety concerns have limited their application in urban environments. In a maritime environment, a drone at risk of crashing can be automatically piloted to a designated area where it can land (or crash) into the sea without injuring life or limb. On land, a safe emergency landing spot is more difficult to find. Droves for delivery of goods to and from floating buildings are more viable at sea than on land.
SEAGRONOMICS
Why do floating farms make sense? It does because (a) the sea has an infinite supply of water, (b) space is abundant and cheap and (c) rain water can be harvested and solar energy can be tapped to desalinate seawater.
Floating farms in freshwater lakes are common. In Inle Lake, Myanmar, floating vegetable and flower gardens have been around since 1960s. There plants are grown on top of natural floating aquatic biomass. Not all is well however. There are lessons to be learnt. The problems at Inle Lake can be overcome at sea.
First, let us look at some basic data. Assuming a particular vegetable (such as tomato, lettuce or cabbage) has a water footprint about 250 litres per kilogram. 1000 kg of the vegetables would require 250,000 litres or 250 cubic metres of water. In tropical areas, the annual precipitation is often in excess of 200 centimetres or 2 cubic metres per square metre. Thus, in order to produce one ton (1000 kg) of vegetable a year one would need a platform with a water catchment area of 125 square metres. To produce 1000 tons of the vegetable, one would need a water catchment of 12,500 square metres or 1.25 hectares. If the precipitation were 100 cm, the area required would be 2.5 hectares; still not an awful lot of space out at sea.
A concrete or polymer float can be designed with a deck suitable for hydroponic culture and below it storage space for rainwater. The design of the float allows rainwater to percolate quickly into the tanks below leaving little time for its evaporation into the atmosphere. The stored water is fed to the plants above it by solar operated pumps. These pumps work more when the sun is shining and therefore is in phase with needs of the plant. Should it be necessary, the water in the tank may be replenished from a nearby freshwater source (a river or lake) or a solar desalination plant (see Fig. 12). Seaweed can be collected and processed to make fertilisers for the floating farms. All post harvesting processes including washing, packing and labelling of the produce could be performed on floating facilities nearby.
The first breakthrough has been made to genetically create plants that are able to grow in seawater. Professor Mark Tester, at the University of Adelaide describe as “promising” that rice may be made salt water tolerant one day. When rice can be grown in the seawater, then large floating platforms would be necessary to enable rice fields to be floating in the sea. And from rice, it may be a short step away to sea-cultivated vegetables, to fruit-trees and trees for timber. Mammoth floating orchards and vegetable gardens may yet be a sight to behold for those alive today. The term seafood would mean more than fishes, crustaceans and molluscs.
FLOATING PARKS AND GOLF COURSES
Parks are essential for the psychological well-being of those living in crowded cities. However, they are land intensive and in very dense cities such as Singapore. Town planners often have to balance the need for recreation with those of housing. Because of the panoramic view, it was quite natural in the early days for town planners to take advantage of it by locating public parks next to waterfronts. However, it is possible now to construct parks floating along the waterfront without compromising their importance or the ecosystem of the waterfront (as would be the case if land were reclaimed to build them). Accessibility is important so they should not be too far away from land to which floating bridges may be linked.
Take the East Coast Park in Singapore as an example. This 185-hectare park occupies a 15-kilometre sea front since the 1970s. The empty sea front stretches out for several kilometres and would have no problem accommodating a cluster of large well-landscaped floating islands with all the current features of the park, including biking and roller blading pathways, beaches and BBQ pits. The potential value of the land that the park currently occupies is several times the cost to build such a park.
Private golf courses in cities are the height of luxury, which only the rich can afford. It is open to question if they are socially justifiable in cities where people live in packed conditions and spend hours in traffic jams. Such poorly utilised land should be freed up for uses that benefit the public at large. Recently, the Singapore Jurong Country Club had to make way for a terminal station for a high-speed rail system from Kuala Lumpur. Another golf club, Keppel Club had been put on notice to vacate. The opportunity is now available for these two clubs to consider (with the blessing of the urban planners) building very exclusive floating golf courses which offer unobstructed views of the ocean as these parks can be located several kilometres from shore since a boat ride is not something beyond the means of their members. In fact, a marina for yachts and speedboats would greatly enhance the club’s prestige. Some ideas and inspiration may come from the first of such floating golf courses in Maldives in the Indian Ocean (see Fig. 11).
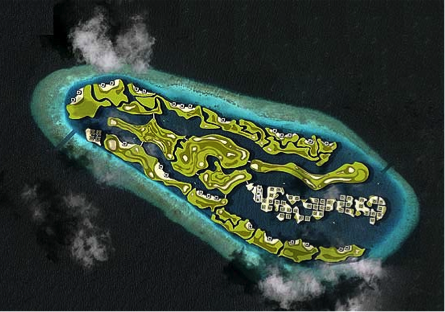
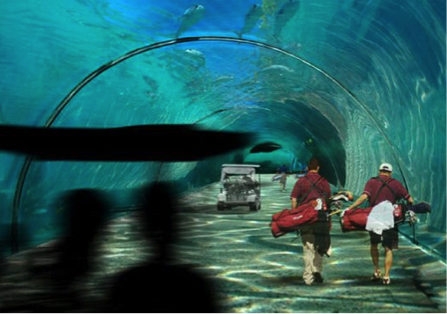
Figure 11: Floating 18-hole golf course interconnecting by underwater tunnels in Maldives
(designed by Dutch Docklands)
The undulating terrain of golf courses is not difficult to create with lightweight structures. These structures easily support the soil, sand, grass trees, streams and ponds. The golf course does not require much buoyancy. Consequently the cost of the float (but not necessarily what is above it!) and its mooring devices would be modest. Water for the vegetation comes from rain (about 20,000 tons of it per hectare per year) and is stored below the course. Power is generated on board. Features not possible in terrestrial clubs but could be considered are underwater restaurants, and recreational areas with glass walls to observe marine life. Dry storage areas for boats can be kept below deck to avoid marring the skyline.
FLOATING SOLAR DESALINATION FARM
Water shortage is a global problem, particularly in Asia and Africa. Without water, life is impossible. With shortage of water, life is harsh. A big part of the lives of villagers is spent collecting water from distant wells and rivers. Their meagre crops die when water supply fails. Without water, sewer systems collapse and diseases spread. On a planet whose surface is 70% covered with water, man in many parts of the world have not yet been able to resolve this basic problem. Current desalination technology is overly complex and too capital intensive for many towns and villages in Asia and Africa.
What is preventing water to be produced by solar energy on a massive scale? To produce clean water by allowing the natural process of evaporation to take place one needs a very large space and a reliable source of raw water. Both are something which crowded cities do not have. If a suitable space were available in the sea, solar desalination would be a viable and a cheap alternative to high-tech solutions such as reversed osmosis. VLFS can provide that space in the sea. Clean water produced can be piped or shipped back to shore.
Figure 12 shows a concept of one possible solution, which also collects rain at the same time. It consists of a VLFS with a trough opened at both ends to the sea. It has several tanks, which are compartmentalised for stability. Over the trough, is a glass dome roof through which the sun’s ray penetrates producing and entrapping a volume of hot air? Air ducts are connected as shown, each with an extraction fan to transport the water-laden vapours into the tanks below where it condenses. The extraction fans lower the pressure in the evaporation chamber and thus accelerating evaporation. To improve the rate of evaporation, the water is agitated by air bubbles. It can be further improved by heating the water using solar collectors (excluded from the illustration for clarity).
For convenience of fabrication, each module may be 10 metres wide and 100 metres long. Ten such modules may be clustered together to produce a catchment farm of one hectare. In many tropical regions, precipitation is about 2 metres a year. This alone will produce 20,000 cubic metres of clean water a year per hectare.
The rate of evaporation at sea depends on a number of factors including the intensity of the sun, the temperature, turbulence and salinity of the seawater, atmospheric pressure and prevailing wind. If we assume evaporation in the chamber can contribute another 10,000 cubic metres per year such a system may produce 30,000 cubic metres (10000 +20000) per hectare of potable water per year.
Four square kilometres (i.e. 400 hectares) would have the potential to produce 12 million (30000 x 400) cubic metres of water yearly under optimum condition. Finding an area of suitable sea space of that size or larger is not a problem for many coastal cities.
The water consumption (including, ablution and sanitation) in third world countries is probably 100 litres/day/person, or approximately 4 cubic metres per person per year. A city of a million people would need 4,000,000 cubic metres per year for domestic use and perhaps twice as much for industrial and commercial uses, depending on the type of its economy. A four square kilometre of floating solar desalination farm would appear to be adequate but even if it is not, it is easy to scale up.
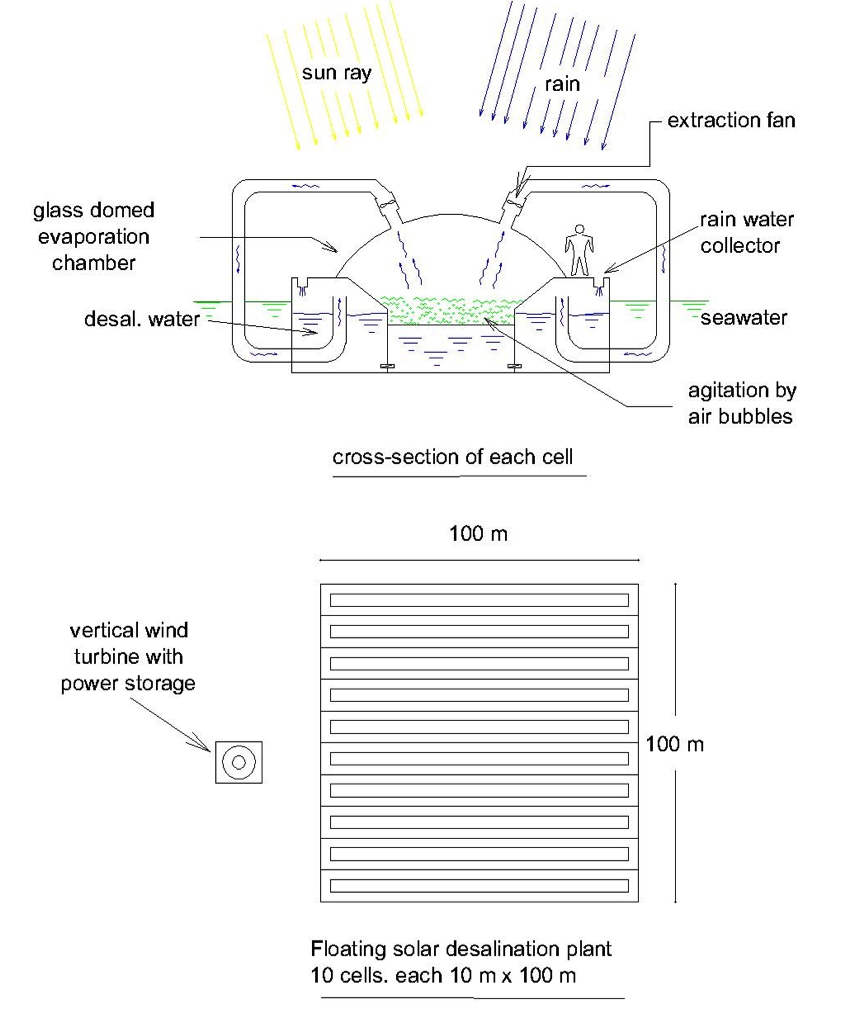
FLOATING ENERGY FARMS
The renewable energy sources that may be derived from the oceans of the world are primarily wind, solar, OTEC (Ocean Thermal Energy Conversion) and algae biomass. Other areas being explored or could be explored include harnessing energy of waves, currents and the bioelectricity of electrogenic sea creature. In such research and development, progress would be enhanced with VLFS technology at hand.
Wind
Energy derived from wind farms has made rapid progress from a global capacity of 47.6 gigawatts in 2004 to 369.6 gigawatts in 2014. Wind farms need vast tracts of land with high prevailing wind. This is hard to find. The ocean has vast space and abundant wind. Consequently, wind farms are trending offshore. Early offshore wind turbines were mounted on foundations sitting on seabed. Such fixed foundations are not economically feasible when water depths exceed 5 to 10 meters. To move further offshore floating foundations are necessary. A literature survey on the research and development of floating wind turbines and farms was made by Wang et al. (2010).
Each wind turbine has its own floating foundation tethered by tension cables. However, for economy of scale a cluster of such floating wind turbines, perhaps 20 to 30 may be mounted on a single platform (see Fig. 13). Vertical axis wind turbines (VAMT), as opposed to horizontal axis wind turbines (HAWT) are better. They function with wind in any direction, have smaller footprints and lower centre of gravity. Given the larger platform and thus much improved stability, the swept area of the blades can be increased by having longer blades over larger diameters. 20 megawatts capacity per turbine is possible. Out at sea, height and noise are not an issue as they are on land.
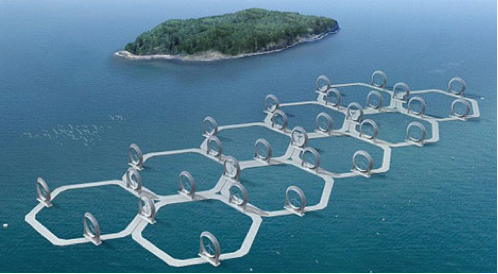
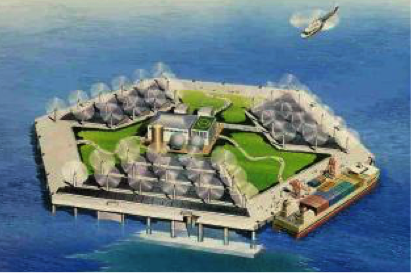
Figure 13: Floating wind farms proposed by the Japanese
Additionally solar panels may be mounted on the platform between the turbines. The VLFS is anchored to keep its position but may be fitted with azipods to enable it to relocate easily to another field in respond to the changing geography of the wind from season to season. They may also respond to market demand, and be deployed to places where electricity tariffs are more attractive.
In the unlimited space of the sea, as many wind turbines as necessary may be installed. More floats can be added incrementally as demand increases. Maintenance crew can be provided with comfortable accommodation on board. It would be easy to unhook and take a platform with such floating turbines to a shipyard for overhaul either under the power of its own azipods or by tugboats. The entire management regime changes going from standalone floating turbines to a group of turbines on a single platform.
Biofuel
Biofuel from seaweed is a proven renewable energy supply. Research conducted at Norwegian University of Science and Technology’s (NTNU) Department of Energy and Process Engineering using hydrothermal liquefaction show that 79 percent of kelp biomass can be converted into bio-oil. This is an amazing. Kelp grows freely in the ocean without human intervention, in massive quantities.
Currently kelp has to be harvested at sea and transported by ship to shore where it is processed in a factory and the oil is stored in tanks. With VLFS, the factory is on board and kelp harvested (they grow at depths less than 30 metres) is processed, where it is collected. The bio-oil is stored in tanks on the same, or in a separate but adjacent, VLFS and may be loaded to ship for export. Besides, if the kelp is depleted and need time to regrow, the floating factory may be relocated to another kelp forest. If the factory requires maintenance or repair it may be towed to a shipyard.
Solar-OTEC integrated system
The development of solar panels has come a long way but it still requires a lot of land area to produce electricity from the heat of the sun incident on the surface of earth. The average solar panel has an output of 150 watts per square metre of panel when the sun is shining on it squarely. In practice, however it is less as the angle of incidence of the sun’s ray is squarely on the panel only briefly each day. The recently completed Solar Star plant in California, USA, has a footprint of 13 square kilometres and generates 579 MW. This translates to an output of 44.5 watts per square metre or a land use of 2.25 hectares per MW. A typical power plant in Asia of 300 megawatt would require a space of almost 7 square kilometres.
Finding 7 square kilometres on land would be quite a task, unless out in the desert. The same or larger area out at sea would not be hard to find. The space out at sea will cost next to nothing compared with an area near an urban environment. The floats on which the solar panels are mounted are inexpensive to construct and to moor, as the panels are light in weight and low in height.
Japan has built a 70 MW floating solar plant in the Kagoshima Prefecture of Southern Japan (see Fig. 14). Called the Kagoshima Nanatsujima Mega Solar Power Plant, it is the largest solar power plant in Japan and it can generate enough electricity to power approximately 22,000 average households. The plant started operation in November 2013.
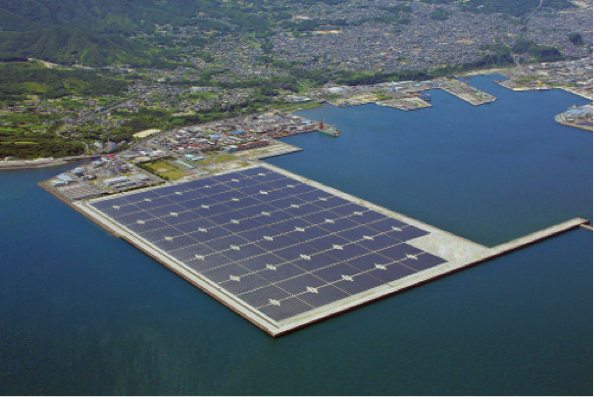
Ocean Thermal Energy Conversion (OTEC) is an established renewable energy technology. It uses the temperature difference between the temperature of the water at the surface and that deep below to produce electricity. The thermal efficiency of the system increases with the magnitude of the temperature difference. With this in mind, we propose that efforts be made to develop a combined cycle of both OTEC and solar panel as illustrated in Fig. 15.
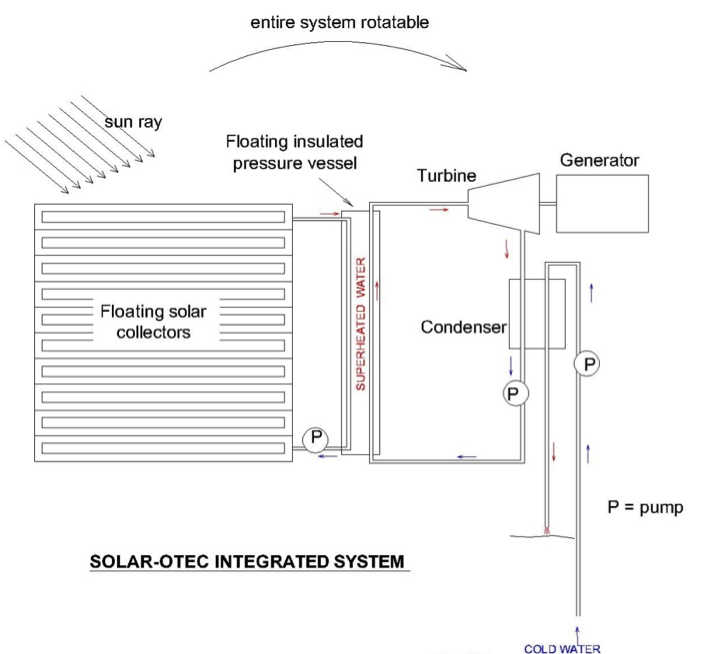
Water circulating in pipes is superheated to more than above 100°C. The superheated water is piped to a floating pressure vessel moored nearby. The heat is transferred to water in a pressure vessel via heat exchangers. This heated water and the cold water of the sea from deep below provide the higher temperature difference for the OTEC cycle. This combined system has not been described before, as far as we can find from research in the Internet and it would be a worthwhile project to pursue.
Another possible means of converting the heat in the pressure vessel into mechanical or electrical engine would be to use the Sterling heat engine.
VLFS THE ENABLING TECHNOLGY
In and of itself, a floating platform does nothing of value for humanity. However, a mega platform floating in the wide and open space of the sea enables man scale up whatever technology he is developing or has developed in dimensions not possible on land. It enables man to reach out to the vastness and the richness of the oceans and often in ways that are more sustainable than what he has developed in the past.
Sustainability is an overused word. It means in our context keeping nature as it is for as long as it is possible. It means living in harmony with Nature. In the last 200 years, man has devastated the environment more than any creature in the past two million years. The land that has supported man has been deforested, the air polluted and the rivers choked with toxic chemicals. Pesticides exterminate insects that pollinate the flowers and grains. Food chains are unwittingly broken. The sea is the last frontier on this planet. Respect it. We may survive another thousand years if we do not repeat the mistakes we make on land. The land we leave behind needs to recover and it can do so if we leave it alone. Nature will do its work, as it does to a trodden path in a forest.
References
Wackernagel, M. (1994). Ecological footprint and appropriated carrying capacity: a tool for planning toward sustainability, PhD Thesis, University of British Columbia, Canada.
Wang, C. M., Watanabe, E. and Utsunomiya, T. (2008). Very Large Floating Structures, Taylor and Francis, Abingdon, UK.
Wang, C.M. and Wang, B.T. (2014). Large Floating Structures: Technological Advances, Springer, Singapore.
Wang, C.M., Utsunomiya, T., Wee, S.C. and Choo, Y.S. (2010). “Research on floating wind turbines: a literature survey,” IES Journal Part A: Civil and Structural Engineering, 3(4), 267-277.
Wang, C. M., Yee, A. A., Krock, H. and Tay, Z. Y. (2011). ‘Research and developments on ocean thermal energy conversion’, The IES Journal Part A: Civil & Structural Engineering, 4(1), 41—52.
Appendix
No more land? Build floating real estate, an article in TODAY dated 14 January 2015
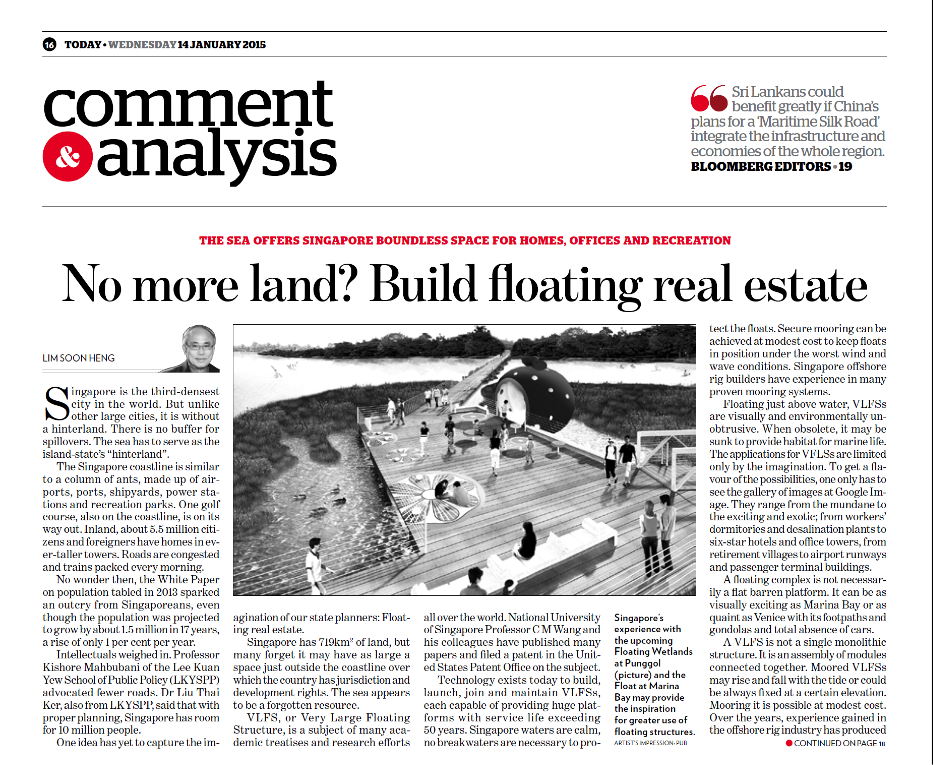
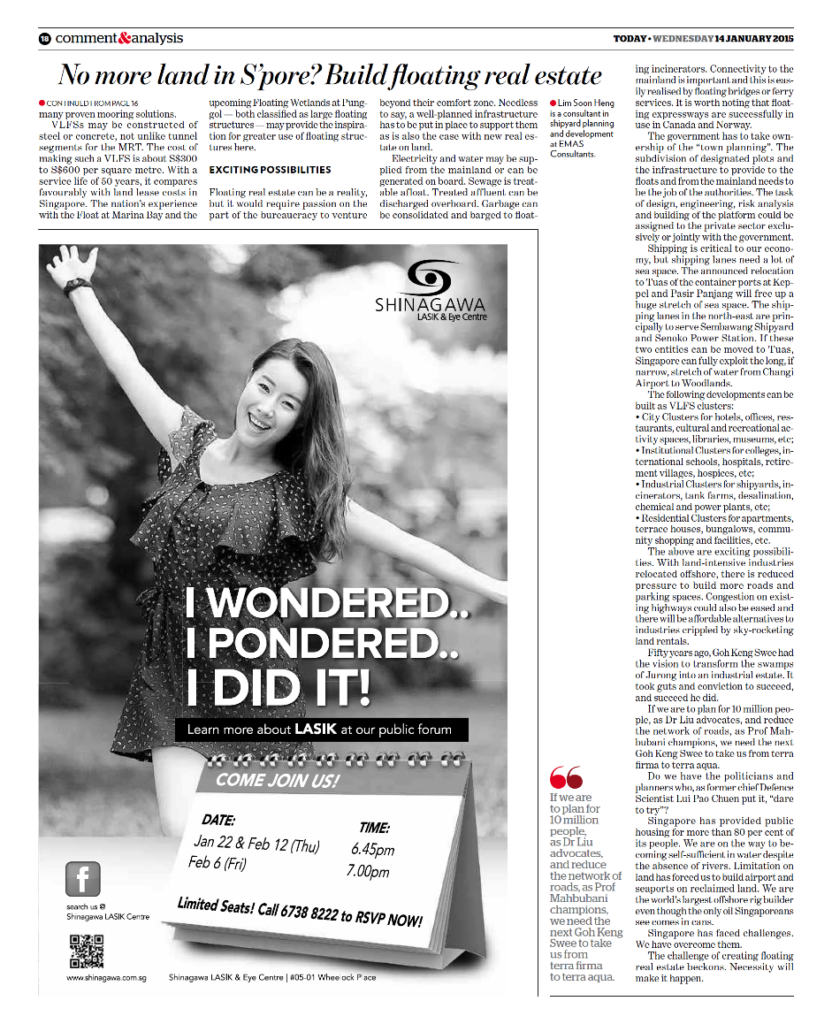